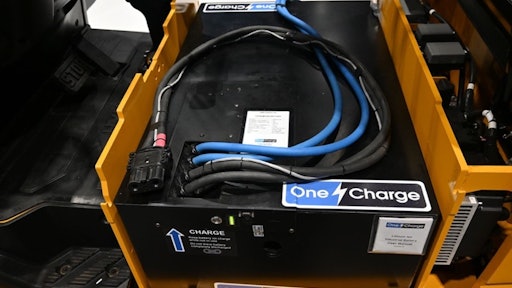
In the fast-evolving world of industrial lithium batteries, extending cycle life— the number of charge and discharge cycles a battery can endure before significant degradation occurs—is one of the key advantages over the incumbent lead-acid technology. A battery management system (BMS) plays a pivotal role in supporting the long cycle life of a lithium battery. Low-priced newcomer battery brands often opt for off-the-shelf BMS solutions with basic functions that are not designed for complex systems. These battery packs fail prematurely, despite using standard-quality lithium cells.
In this article, we explore the significance of a robust BMS, the impact of battery cell chemistry on cycle life, and how a comprehensive product life-cycle infrastructure contributes to making batteries last longer.
Product life-cycle infrastructure plays an important role in extending a battery's useful life. It may include professional support with setup, individual settings, and integration with a charger and host vehicle at installation; regular service; reliable and complete data collection for automated troubleshooting; access to data and predictive maintenance; and accurate battery specifications logging.
Crucial Role of Battery Management Systems (BMS)
The battery management system (BMS) plays a pivotal role in the efficient operation and longevity of industrial batteries. At its core, the BMS controls power output and serves as the guardian of battery health, overseeing critical functions that directly impact performance and safety. One of its fundamental tasks is to regulate and optimize the charging and discharging processes and equalize the performance of individual cells, preventing overcharging or deep discharging that can lead to capacity degradation or even safety hazards. Moreover, the BMS constantly monitors and maintains a suitable temperature range inside the battery pack, mitigating the risk of thermal runaway and ensuring that the battery operates optimally.
Integrating a forklift battery with a control area network (CAN) guarantees that the battery and the host truck or charger are working as one system and exchange all necessary data. Advanced forklift batteries may feature multiple CAN connections as part of their BMS. Some manufacturers have developed a multi-CAN BMS that connects the battery, the truck, the charger, and all the internal elements and components of the battery pack itself. Other connections are reserved for outside equipment, like an outside battery discharge indicator (BDI).
The significance of a reliable, advanced multi-functional BMS becomes evident when considering the consequences of buying a battery pack equipped with an off-the-shelf BMS with limited capabilities, used by cheaper battery brands to cut corners. Such generic BMS implementations lack the sophistication needed to cater to specific battery chemistry and equipment application. As a result, batteries paired with a subpar BMS may suffer from compromised cell performance, reduced capacity, and shortened lifespan. Additionally, safety concerns escalate as these rudimentary BMS may struggle to detect and manage potential issues, increasing the likelihood of failures.
In essence, the battery management system stands as the bulwark between seamless battery performance and potential setbacks. Its intricate orchestration of charging, discharging, communication, and temperature control not only ensures efficient operation but also safeguards against premature wear and safety hazards, making it an indispensable component of battery technology.
Unleashing the Potential: Battery Cell Chemistry vs. Engineering Design
The chemistry of a cell, the fundamental battery building block, establishes the upper threshold for a battery's cycle life. Two prominent contenders in the energy storage arena, lithium iron phosphate (LFP) and lithium nickel manganese cobalt oxide (NMC), along with traditional lead-acid counterparts, exemplify this chemistry-dependent variability of a battery’s cycle life.
Traditional flooded lead-acid batteries deliver around 1500 cycles before reaching the end-of-life stage when they lose capacity and can’t keep a charge anymore. LFP and NMC battery cells promise from 3000 to 7000 cycles, and beyond. While NMC batteries offer higher energy density and slightly higher voltage, LFP batteries excel in longevity.
But battery chemistry alone does not dictate cycle life; engineering design plays an equally crucial role. For example, a cell-to-pack LFP battery can outperform NMC at a pack level, even though at a cell level in laboratory conditions NMC cells demonstrate higher numbers both in longevity and energy density. Battery design can mitigate the impact of extreme temperature, uneven cell aging, and stress-induced degradation, thereby enhancing the overall longevity of the battery pack. Moreover, a well-engineered battery system with robust thermal management, efficient current distribution, and optimal electrode configurations will extend the number of warrantied cycles and years of service.
The Holistic Approach: Product Life-Cycle Infrastructure
Modern advanced industrial batteries are complex devices and like any equipment of this level, they require professional support at various stages. The process begins with the pre-delivery inspection (PDI) of batteries, where expert guidance ensures seamless integration with both the charger and the host vehicle. The correct settings guarantee optimal performance and minimize potential glitches.
The data capabilities of modern lithium batteries allow for remote predictive maintenance, which not only safeguards against failures but also extends the useful life of the battery. The implementation of reliable data collection mechanisms helps to troubleshoot the battery pack remotely. This data-driven approach empowers timely interventions, averting potential breakdowns and downtime, and optimizing performance.
A trained technician can usually resolve the majority of common problems with battery performance in minutes, which is why battery manufacturers invest in training programs for their partners. A few hours spent on training may save days of downtime and improve equipment utilization dramatically.
Armed with insights into real-time battery health and performance metrics, facility and warehouse managers can make calculated choices to enhance efficiency and minimize operational disruptions. At the base of this data-centric paradigm lies the accurate logging of battery specifications. With automated recording and updating of the model codes and parameters of all battery components, technicians can make sure any issue with battery charging or performance can be diagnosed and resolved efficiently.
In summary, a lithium battery's longevity is a result of multiple factors working in parallel. A robust battery management system (BMS) is essential to ensure optimal battery performance and avoid premature failure. While cell chemistry sets the limit for cycle life, engineering design plays a significant role in defining the battery's warrantied years of service. A comprehensive product life-cycle infrastructure, including professional support, regular service, data collection, and access, enhances a battery's life and contributes to sustainable and efficient motive power. By understanding and addressing these critical factors, we can unlock the full potential of lithium batteries and further improve the efficiency of energy storage.