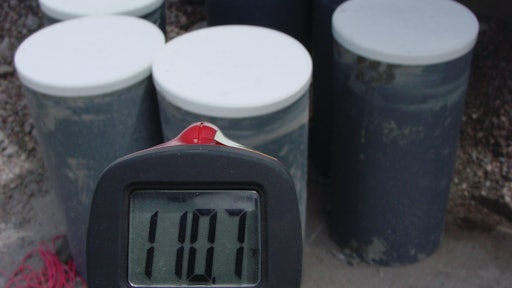
Concrete must gain adequate strength to carry its weight and construction loads before removing formwork, reshoring or backshoring. Engineers frequently specify minimum in-place concrete strengths before contractors can post-tension, backfill against walls, open pavements to traffic or terminate protection during cold weather. For these reasons, contractors must know how to properly estimate in-place concrete strengths for recently placed concrete, especially during cold weather. Otherwise, worker safety and the quality of the structure may be jeopardized.
Field-cured test cylinders and maturity factors are often used for estimating in-place concrete strengths. However, testing field-cured cylinders is the default procedure specified by the building code. Other methods — including maturity factors and cast-in-place cylinders for slabs, penetration resistance, and pullout strength — require approval by the architect/engineer and may require approval by the building official.
Temperature and time
Strength gain of concrete depends on a combination of curing temperature and time. The rate of hydration, or the chemical reaction between cement and water, is a function of the concrete temperature. As the concrete temperature increases, the rate of hydration and resulting strength gain increases. Conversely, the rate of strength gain decreases as the concrete temperature decreases. For this reason, delayed concrete strengths are common during cold weather unless contractors implement precautions. Of course, concrete strength increases with time if there are adequate curing conditions to promote hydration.
Field-cured test cylinders
Standard and field curing are different procedures specified by ASTM C31 for curing concrete test cylinders. Standard-cured test cylinders, sometimes referred to as lab-cured cylinders, represent ideal or nominal concrete strengths. Temperature ranges for standard curing are 60° F to 80° F for a period up to 48 hours (initial curing) and 73.5 ± 3.5° F for the balance of the 28-day cure period (final curing) for concretes with specified strengths up to 6,000 psi. Concrete with specified strengths of 6,000 psi or greater must follow the tighter temperature range of 68° F to 78° F for initial curing. For standard curing, temperatures and times are standardized to produce uniform curing conditions. That’s why strengths derived from standard-cured test cylinders are used for concrete strength acceptance.
Field curing is different than standard curing. It consists of storing test cylinders as near as possible to the in-place concrete and protecting cylinders from the elements in the same way as the in-place concrete. Curing conditions for the test cylinders should be the same as the curing conditions of the in-place concrete. By subjecting the test cylinders to the same temperature-time history as the in-place concrete, it is presumed the strength of the test cylinders represents the in-place concrete strength.
Field-cured test cylinders typically underestimate the true in-place concrete strength because of the thermal mass of a test cylinder (4 in. x 8 in. or 6 in. x 12 in.) as compared to the significantly larger thermal mass of the concrete element represented. Typically, curing temperatures for the test cylinders are less than the actual in-place concrete temperatures, even when test cylinders are tucked under the curing blanket and stored adjacent to the concrete represented.
Strengths obtained from field-cured test cylinders are typically conservative. However, field-cured cylinders may grossly overestimate the in-place concrete strength if the fielded-cured cylinders are stored and cured in the job trailer.
With few exceptions, standard-cured cylinder strengths are greater than field-cured cylinder strengths because standard curing temperatures create faster rates of hydration and strength gain than typical field curing temperatures. For this reason, always use standard-cured cylinder strengths for concrete strength acceptance. More important, use only field-cured cylinder strengths to make construction decisions such as determining when to remove formwork and shoring, begin post-tensioning or determine when to place a structure into service. Never use standard-cured test cylinders in lieu of field-cured test cylinders. Failing to properly estimate in-place concrete strengths can jeopardize worker safety and may damage the structure.
Maturity method
The maturity method (ASTM C1074) is more accurate, reliable and economical for estimating in-place concrete strengths than field-cured test cylinders. It is based on the concept that concrete temperature and curing time is directly related to concrete strength. Specifically, this method uses a pre-established temperature-time-strength relationship for a given concrete mixture to estimate in-place concrete strengths.
Steps to estimate in-place concrete strengths using the maturity method include:
1. Prepare at least 15 laboratory test cylinders and embed temperature probes into at least two of the cylinders for a given concrete mixture, cure at room temperature and compute maturity factors M(t) for various elapsed times corresponding to strength tests using the following equation:
M(t) = SUM(Ta minus To)Δt
where:
M(t) = maturity factor at age (t), degree–hours, ° F–h
Δt = time interval, hrs
Ta = average concrete temperature during time interval (Δt), ° F
To = temperature below which no strength increase occurs, ° F (14° F to 32° F)
Then establish a smooth strength-versus-maturity curve by plotting computed maturity factors M(t) versus corresponding concrete strengths.
2. Measure the temperature-time history of the in-place concrete by embedding temperature probes at critical locations as defined by the severity of concrete exposure and loading conditions.
3. Read the temperature-time data and calculate the maturity factor for the elapsed time of the in-place concrete using the maturity factor M(t) equation. Modern maturity equipment automatically computes and records maturity factors.
4. Estimate the in-place concrete strength by entering the pre-established strength-versus-maturity curve with the computed M(t) for the in-place concrete and read the estimated strength as shown in Figure 1. Again, this step is typically performed automatically with today’s modern maturity equipment and software.
Example
Due to an approaching cold front, a contractor installed temperature probes into a wall placed at 9:00 a.m. September 1. The concrete supplier provided a maturity-versus-strength curve for the concrete being used as shown in Figure 1. Specifications for the project required a minimum concrete strength of 3,000 psi before placing and compacting backfill against the wall.
As shown in Table 1, elapsed times and in-place concrete temperatures were recorded in Columns 2 and 3 for the dates shown in Column 1. Using Column 3, average in-place concrete temperatures were computed and recorded into Column 4. Next, the contractor subtracted 23° F, or the temperature where strength gain essentially stops from the average temperatures shown in Column 4, and entered the adjusted temperatures into Column 5. Elapsed times in hours from Column 2 were computed and entered into Column 6. The contractor then multiplied the temperatures in Column 5 times the elapsed times in Column 6 and entered the values (° F-h) in Column 7. For Column 8, the cumulative maturity factors were computed and entered for the different elapsed times.
Finally, the contractor entered the pre-established strength-versus-maturity curve (Figure 1) provided by the concrete supplier with the in-place cumulative maturity factors from Column 8 and read the corresponding in-place concrete strengths. The estimated in-place concrete strengths were entered into Column 9 (e.g. for an in-place 5,070 ° F-h maturity factor, the corresponding concrete strength was found to be 3,100 psi from Figure 1).
Since the specifications required a minimum 3,000 psi concrete strength to ensure adequate wall strength for installing the backfill, the contractor must wait until the concrete has achieved at least a 5,000 ° F-h maturity factor. To shorten the cure period, the contractor can use hot water for batching, add a chemical accelerating admixture to the concrete or add additional insulation blankets so more heat can be generated and maintained.
Limitations
Erroneous strength estimates can occur if the in-place concrete is significantly different than the concrete used to develop the pre-established temperature-time-strength curve. Changes in materials, water and air contents, and batching accuracy can introduce strength estimation errors. ASTM C1074 recommends supplementary testing be performed to periodically verify the temperature-time-strength curve, especially when life-threatening construction activities are based on estimated in-place concrete strengths.
References
ACI306R-10 Guide to Cold Weather Concreting, American Concrete Institute, www.concrete.org, Mindness, S., Young, J.F, and Darwin, D., Concrete, 2nd Edition, Prentice Hall, 2003.
Kim Basham, PhD, P.E. FACI is president of KB Engineering LLC, which provides engineering and scientific services to the concrete industry. Basham also teaches seminars and workshops dealing with all aspects of concrete technology, construction and troubleshooting. He can be reached via email at [email protected].
Methods for Estimating In-place Concrete Strength
Here are several alternatives to field-cured test cyliners for estimating in-place concrete strength.
ASTM C31/C31M-12 Standard Practice for Making and Curing Concrete Test Specimens in the Field - Described in this article.
ASTM C873/C873M-10a Standard Test Method for Compressive Strength of Concrete Cylinders Cast in Place in Cylindrical Molds - Involves casting in-place test cylinders into slabs, only for with depths between 5 and 12 inches.
ASTM C803/C803M-03(2010) Standard Test Method for Penetration Resistance of Hardened Concrete - Involves shooting pins into the concrete with a power-actuated tool and measuring pin penetration.
ASTM C900-06 Standard Test Method for Pullout Strength of Hardened Concrete - Requires placing bolts into formwork before placing concrete.
ASTM C1074-11 Standard Practice for Estimating Concrete Strength by the Maturity Method - Described in this article.